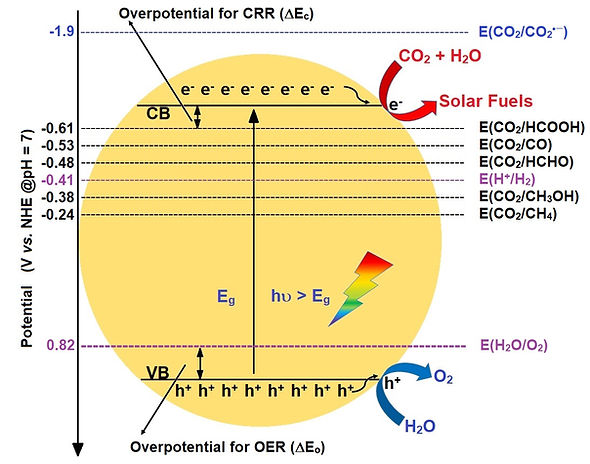
In recent times, the graphitic carbon nitride (g-C3N4) has received substantial recognition as a photoactive semiconductor material having interesting attributes favourable for artificial photosynthesis and optoelectronics applications. It is a metal free semiconductor which is highly tuneable in terms of its optoelectronic properties but most importantly it is environmentally benign. The relatively low bandgap of this material (~2.7 eV) allows it to absorb a good number of photons in the visible domain of the solar spectrum which is the most important criterion for an effective artificial photosynthesis catalyst. The better absorption of photons in the visible region leads to better utilization of the solar spectrum resulting in an enhanced generation of photoinduced charges which take part in different photoredox processes, thus leading towards higher conversion efficiencies. Despite the material having a wide array of interesting physicochemical and electronic properties useful for photocatalytic and light harvesting applications, the real-life applicability is still hindered because of some of the shortcomings of pristine g-C3N4. The bulk g-C3N4 suffers from rapid recombination of excitons, low electrical conductivity, insolubility in most common solvents and most importantly poor absorption of light above 460 nm. The modulation processes of improving the physicochemical attributes of the photocatalyst entities came a long way in diversified dimensions and in the case of carbon nitride-based materials, it is not an exception. Modifications in geometric architecture, electronic structure, introduction of elemental doping agents and adaptation of defect engineering process are some of those basic exploitable techniques which can boost the overall photocatalytic performance of the photocatalysts. However, techniques like doping with metallic and non-metallic elements, surface functionalization and architecture alteration are not quite effective as the molecular modification or molecular doping strategy as it directly augments the physicochemical and photophysical properties of g-C3N4 by tweaking the π- electron delocalization. Directly altering the π-electron cloud of the carbon nitride surface can massively affect the electronic structure of the system which in turn can lead to superior light absorption and charge carrier dynamics. The carbon nitride framework is an extended
structure of N-bridged tri-s-triazine rings. The carbon and nitrogen atoms in the motif are sp2 hybridized and the overall structure possesses a π-electron cloud delocalized all over the 2D framework. Thermal copolymerization of nitrogen rich precursors like melamine, urea and dicyandiamide has been a well-established route for the synthesis of pristine carbon nitride. In the course of the reaction, addition of some aromatic or π-conjugated molecules other than the original carbon nitride precursors can cause freezing or locking of these new π-delocalized building blocks in some places of the carbon nitride framework, disrupting the original translational symmetry of the 2D framework. The presence of these non-triazine building blocks in the framework greatly affects the nature of electronic delocalization of the π-electron cloud, leading towards significant alterations in the chemical composition and overall electronic band structure of the carbon nitride materials. As compared to functional side group based modification, organic molecular modification exhibits more pronounced effects in terms of electronic property alteration of g-C3N4 owing to the fact that, unlike side group modification which perturbs the surface of the 2D structure of the material, molecular modification perturbs the framework of the material directly, further altering the nature of the π-delocalization itself.

The long term motive of our work is to synthesize a noble metal free and environmentally benign photoactive nanocomposite comprised of an engineered polyoxometalate sandwiched in between modified carbon nitride and N-doped GO which can act as a potent photocatalyst for the photochemical production of clean fuel in a mimetic artificial photosynthesis type of reaction where water can be split into its constituent elements H2 and O2 (though O2 is less preferred and can be suppressed via using sacrificial donors), keeping in mind that H2 is having a great potential as a clean fuel. By tuning the band structure, CO2 photoreduction to methanol or methane is also quite possible by a similar system. The use of noble metals can be bypassed by using carefully designed first row transition metal containing polyoxometalate structures which are a cheaper and safer option as these metal ions are often encountered in biochemically crucial enzymatic environments. Overall, the research aims for the synthesis, characterization and validation of environmentally benign, robust and highly active catalyst library based on nature’s own chosen set of precursors and materials for a highly effective mimetic Z-scheme artificial photosynthesis type application
